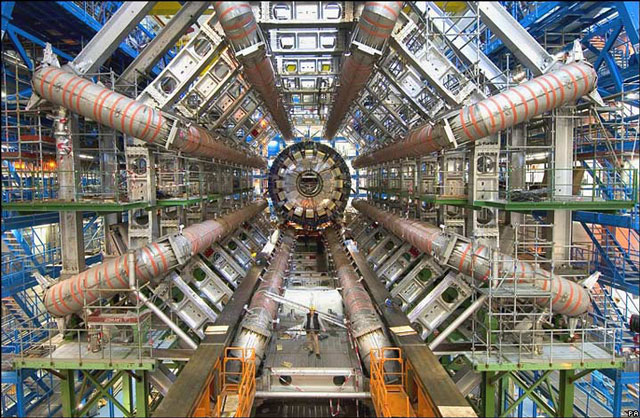
Wits University scientists are excited about getting closer to understanding “dark matter”, the institution said on Wednesday.
The High Energy Physics Group (HEP) at Wits recently established the “Madala hypothesis”, in describing a new boson — or subatomic particle — named the “Madala boson”.
They did this using data from experiments that led to the discovery of the Higgs boson at the European Organisation for Nuclear Research (Cern) in 2012.
The experiment was repeated in 2015 and 2016, after a two-and-a-half year shutdown of the Large Hadron Collider (LHC) at Cern.
“Based on a number of features and peculiarities of the data reported by the experiments at the LHC and collected up to the end of 2012, the Wits HEP group, in collaboration with scientists in India and Sweden, formulated the Madala hypothesis,” said Bruce Mellado, HEP team leader.
The Wits Madala project team consists of approximately 35 South African and African students and researchers who are contributing to understanding data from the LHC experiments.
This is along with phenomenological investigations from theorists such as Alan Cornell and Mukesh Kumar and support in the area of detector instrumentation from Elias Sideras-Haddad, all of Wits University.
The hypothesis describes the existence of a new boson and field, similar to the Higgs boson.
However, where the Higgs boson in the standard model of physics only interacts with known matter, the Madala boson interacts with dark matter, which makes up about 27% of the universe.
The theory that underpins the understanding of fundamental interactions in nature in modern physics is referred to as the standard model.
With the discovery of the Higgs boson at the LHC in 2012, the standard model is now complete. The discovery earned Peter Higgs and Francois Englert the Nobel Prize in Physics in 2013.
However, this model is insufficient to describe phenomena such as dark matter.
The universe is made of mass and energy. The mass that we can touch, smell and see, the mass that can be explained by the Higgs boson, makes up only 4% of the universe’s mass-energy budget.
The rest of the mass in the universe is simply unknown, yet it makes about 27% of the world around us.
The next big step for the physics of fundamental interactions now is to understand the nature of dark matter: What is it made of? How many different types of particles are there? How do they interact? How does it interact with the known matter? And what can it tell us about the evolution of the universe?