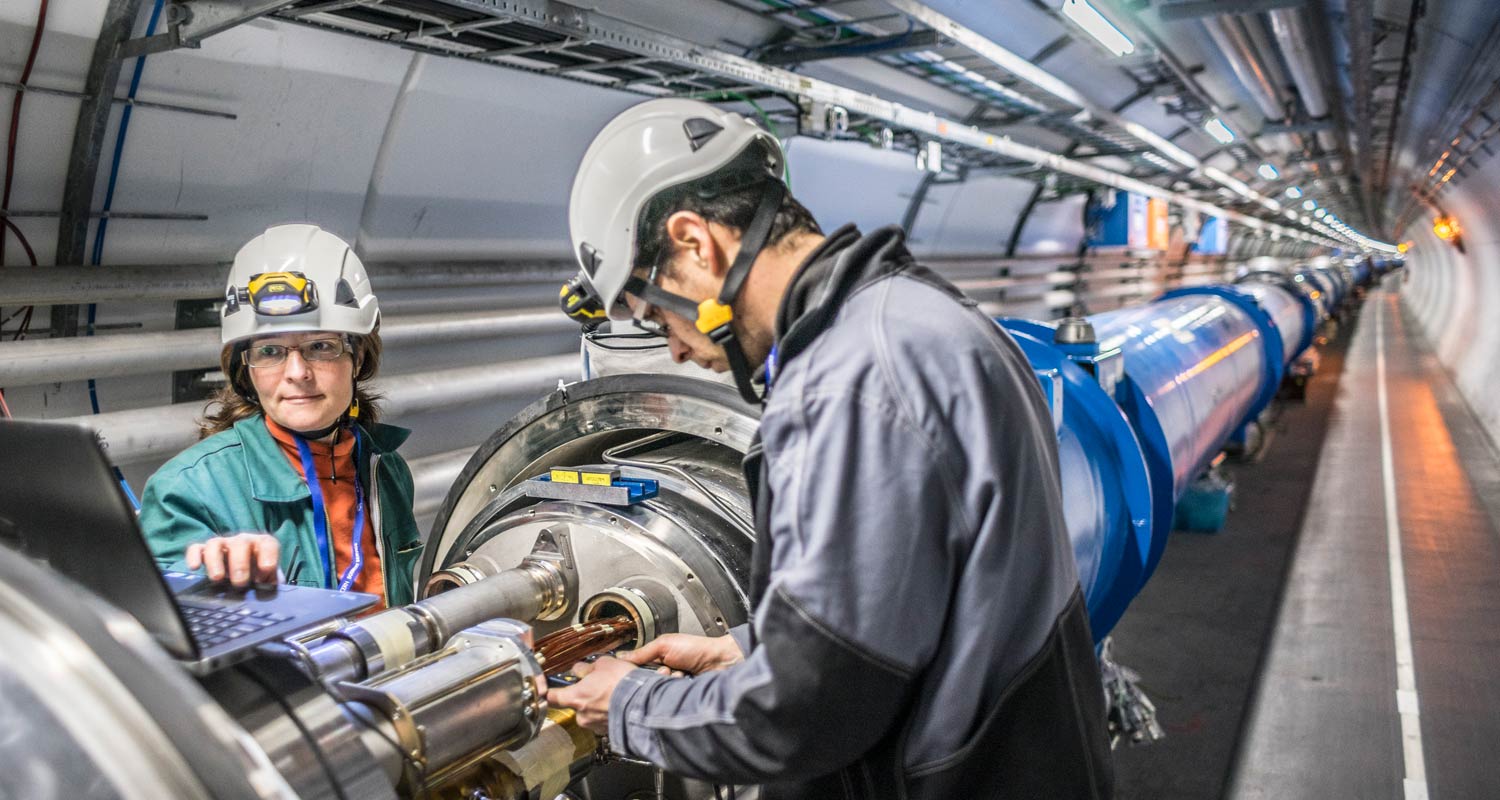
Why do particles have mass? That was a question that preoccupied the late Nobel Prize-winning physicist Peter Higgs. His proposed explanation sparked a half-century quest, which culminated in the detection of the boson that bears his name in 2012.
That discovery, by scientists at Cern’s Large Hadron Collider, completed the standard model of physics, which describes how particles behave and fundamental forces interact. It was an experimental triumph with loads of ancillary benefits. But it may also have marked the zenith of a particular approach to research: building ever-larger particle accelerators.
With the LHC reaching the end of its useful life around 2040, Cern, the European Organisation for Nuclear Research, is planning for a massive successor that would allow for higher-energy experiments. The Future Circular Collider, as it’s known, would require digging some 90km of tunnel under the Swiss and French countryside, over three times the length of the LHC, at an estimated cost of more than US$17-billion for its initial phase and perhaps another $50-billion for the final collider, which wouldn’t be ready until the 2070s. Expect the costs to rise and deadlines to lengthen.
To be sure, plenty of mysteries remain to be solved. Some 95% of the universe is composed of dark matter and dark energy, neither of which is well understood. New particles may yet await discovery. The Higgs boson itself requires further study. But the FCC would be built without the kind of road map that Peter Higgs provided for its predecessor. Finding new physics may take even higher energy scales or lie beyond the limitations of new colliders. Given the generational time frames and costs, that’s a formula for spinning wheels.
Many other promising experiments are underway, both to probe the energy frontier and to look for physics beyond the Standard Model, from ambitious new research on neutrinos to telescopes that measure the universe’s earliest signals. Scientists in the US are exploring building a muon collider, which would (if technical obstacles can be overcome) achieve cleaner collisions at higher energies with a smaller footprint. A collider using plasma wakefield technologies shows similar potential.
Smaller projects
It’s equally important to pursue smaller projects. A quieter announcement from Cern in March — about the Search for Hidden Particles experiment, or SHiP — offers one example. SHiP will direct excess high-intensity proton beams from Cern’s Super Proton Synchrotron accelerator at a fixed target, filtering out conventional particles to detect hidden ones that interact weakly with ordinary matter. At about €100-million, its costs are reasonable. And it shouldn’t take a lifetime to build; designers expect to collect data by 2030. If SHiP finds something, it could be the ultimate bang-for-the-buck investment, leveraging existing tools to solve some of the most persistent problems of the field.
Funding physics remains eminently sensible. In addition to advancing scientific knowledge and sustaining a skilled workforce, such research has spawned a slew of useful inventions, including medical-imaging technologies, cancer therapies, advanced computing networks, radiocarbon dating, superconducting magnets and the world wide web. It is also helping to explain the deepest truths of the universe, which should count for something.
But building ever-bigger particle colliders looks likely to have diminishing returns and could well crowd out other worthy efforts. That risk may lessen in time: new techniques could improve on current technology, or theoretical advances may offer a new road map for exploration. But for now, Europe’s leaders need to carefully weigh costs and benefits, and be open to other approaches to solving the riddles of existence. — (c) 2024 Bloomberg LP